Outrageous Tips About Does Increasing Tr Increase Snr
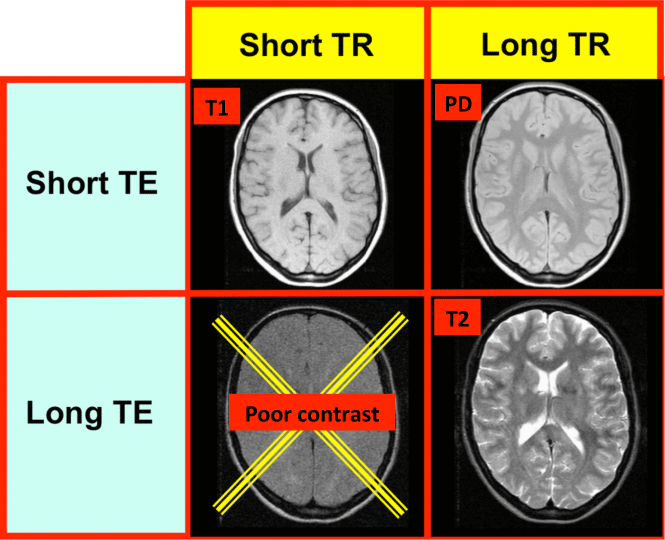
Peering into the Signal's Clarity: Does Repetition Time Really Boost Signal-to-Noise? Let's Investigate
Laying the Groundwork: Signal and Noise Demystified
Ever ponder how we capture those detailed images or crystal-clear audio transmissions? At its core lies a delicate balance between the information we truly seek (the 'S', or signal) and the unwanted background interference (the 'N', or noise). Their relationship, quantified as the Signal-to-Noise Ratio (SNR), is a vital measure across diverse fields, from your mobile phone reception to the intricate world of medical scans. A higher SNR generally equates to a more distinct and dependable signal. Now, where does 'TR' fit into this puzzle? Predominantly encountered in Magnetic Resonance Imaging (MRI), TR, or Repetition Time, signifies the time interval between successive bursts of radio waves directed at the patient. It's a fundamental setting that influences image quality and the types of tissues we can effectively visualize. So, the million-dollar question: does adjusting this TR setting directly enhance our SNR?
Think of trying to hear a friend speaking at a bustling cafe. Your friend's voice is the signal, and the surrounding chatter is the noise. A better SNR would be akin to your friend speaking louder or the cafe becoming quieter, making their words easier to comprehend. Now, consider TR as how frequently your friend repeats key phrases. Does repeating these phrases more often (a shorter TR) inherently make their voice louder relative to the surrounding din? Not necessarily. It's more about how those repetitions interact with the acoustics of the cafe and your ability to focus on their voice. Similarly, in MRI, TR influences the magnetic behavior of the tissues being examined, which in turn affects the strength of the signal we ultimately detect.
It's not a straightforward cause-and-effect scenario where simply increasing one automatically elevates the other. The interaction between TR and SNR is more intricate and heavily reliant on the specific context and the underlying physical principles. For instance, in MRI, a longer TR allows for a more complete recovery of the magnetic alignment within the tissues, potentially leading to a stronger signal. However, this also requires more time, which isn't always ideal in a clinical setting. Conversely, a shorter TR enables faster image acquisition but might compromise the signal's strength. It's a delicate balancing act, a careful optimization to achieve the best possible image quality within the practical limitations of time and patient comfort. So, while TR undeniably plays a significant role in the overall imaging process and indirectly influences SNR, it's not a direct, one-to-one correlation.
Therefore, stating unequivocally that "increasing TR increases SNR" would be an oversimplification of a more complex reality. The relationship is far more nuanced, involving the inherent properties of the tissues being imaged, the specific design of the pulse sequences used, and the ultimate goals of the diagnostic examination. We need to delve deeper into the underlying mechanisms at play to truly grasp how manipulating TR affects the clarity and reliability of our signals. It's akin to fine-tuning a musical instrument; each adjustment has an impact, but the final harmony depends on the interplay of all the settings. Let's explore some of these fundamental mechanisms in greater detail.
The Magnetic Tapestry: How TR Shapes Signal Intensity in MRI
Dissecting the Underlying Processes
Within the fascinating realm of MRI, the signal we detect originates from the behavior of protons, the tiny magnetic components within the body's tissues. These minuscule magnets align themselves with a powerful magnetic field. When a burst of radio waves (an RF pulse) is applied, these aligned protons absorb energy and are momentarily knocked out of their aligned state. Once the RF pulse ceases, the protons gradually return to their original alignment, a process known as relaxation. This relaxation process emits a signal that is picked up by the MRI scanner. Now, TR enters the picture as the time interval between these successive RF pulses. Its duration significantly affects how much the protons have realigned with the magnetic field before the next pulse arrives.
A longer TR provides more time for this realignment, or longitudinal magnetization recovery, to occur. Imagine it like stretching a spring. A longer time allows the spring to fully return to its original length, ready for the next stretch. Similarly, with a longer TR, more protons have had sufficient time to realign with the magnetic field, resulting in a stronger signal when the subsequent RF pulse is applied. This stronger signal, in turn, can contribute to a higher SNR, assuming the background noise levels remain relatively constant. However, this advantage comes at the expense of longer scan durations, which can be a significant consideration in real-world clinical practice.
Conversely, a shorter TR means that the next RF pulse is applied before the protons have fully recovered their longitudinal magnetization. This leads to a weaker signal. Picture trying to stretch the spring again before it has fully returned to its resting state; the subsequent stretch will be less forceful. Similarly, a shorter TR results in less longitudinal magnetization available to be influenced by the next RF pulse, yielding a weaker signal. While this allows for faster imaging, the trade-off is often a lower SNR. This can manifest as images with more graininess or artifacts, making it more challenging to discern subtle details that might be crucial for diagnosis.
Therefore, the relationship between TR and signal intensity is not a simple linear one. It's governed by the T1 relaxation time of the specific tissues being imaged. T1 is an inherent property of each tissue that describes how quickly its longitudinal magnetization recovers. To maximize signal intensity for a particular tissue type, the TR should ideally be somewhat comparable to its T1 relaxation time. Using a TR significantly shorter than T1 will lead to signal saturation, while using a TR much longer than T1 will unnecessarily extend the scan time without a substantial gain in signal. It's a delicate balancing act, carefully tailored to the specific diagnostic question and the tissues of primary interest.
The Unseen Adversary: Understanding Noise in the SNR Equation
Identifying the Source of Interference
While we've primarily discussed how TR influences the 'S' in SNR, the 'N' is an equally critical component. Noise in MRI can originate from various sources, both within and outside the patient and the scanner itself. Thermal noise from the electronic components, radiofrequency interference from external devices, and even physiological noise arising from the patient's body movements can all contribute to degrading the quality of the resulting MR image. A thorough understanding of the sources and characteristics of this noise is essential when considering how manipulating parameters like TR ultimately affects the overall SNR.
Interestingly, while TR's primary impact is on signal strength, it can indirectly influence the noise characteristics in certain situations. For example, the longer scan times associated with longer TRs might potentially increase the susceptibility to specific types of physiological noise, such as artifacts caused by patient movement. If a patient moves more during a prolonged scan, this can introduce unwanted signals that effectively increase the noise level in the image. Conversely, shorter TRs and faster scans might reduce the impact of motion artifacts but at the cost of a lower intrinsic signal strength.
Furthermore, the receiver bandwidth, another important adjustable parameter in MRI, can also play a role in the level of noise captured. A wider receiver bandwidth allows for faster data acquisition but typically samples a broader range of frequencies, which can include more noise. The choice of TR can sometimes be linked to the chosen receiver bandwidth, further complicating the direct relationship between TR and SNR. It's an intricate web of interconnected parameters, each influencing the final image quality in its own unique way. Optimizing SNR involves not only maximizing the signal we want but also minimizing the contribution of unwanted noise.
Therefore, when evaluating the impact of TR on SNR, we must not disregard the noise component. Simply increasing TR might boost the signal, but if it simultaneously exacerbates noise due to longer scan times or necessitates a wider receiver bandwidth, the overall SNR might not improve as anticipated, or could even decline. A comprehensive approach to image optimization involves carefully considering the interplay of all relevant parameters to achieve the best possible balance between the desired signal and the inherent noise for the specific clinical application at hand.
Echoes Across Disciplines: TR's Analogues and SNR Beyond MRI
Parallel Concepts in Other Scientific Fields
While the specific term 'TR' is largely confined to the domain of MRI, the fundamental principle of adjusting the timing of repeated measurements or excitations to optimize the desired signal relative to background noise resonates across numerous other scientific and engineering disciplines. Consider, for instance, in radar systems, the pulse repetition frequency (PRF) bears a conceptual similarity to the inverse of TR. The PRF influences the maximum measurable range and velocity, and also affects the average power transmitted, which in turn impacts the strength of the returned signal and consequently the SNR of the detected echoes.
In the realm of audio signal processing, the repetition rate of specific patterns or the duty cycle of pulsed signals can be manipulated to enhance the desired frequencies and minimize unwanted background sounds. Techniques such as noise gating rely on identifying periods where the signal is dominant and suppressing the output during periods of low signal and high noise. While not a direct equivalent of TR, the precise timing of signal acquisition and processing is crucial for improving the perceived SNR in audio recordings and transmissions.
Even in the field of communication systems, the symbol rate and the precise timing of signal transmission are critical for ensuring reliable data transfer in the presence of interference. Adjusting these parameters can influence the power spectral density of the transmitted signal and its susceptibility to external noise sources. Error correction codes, which introduce a degree of redundancy into the transmitted data, can be viewed as a method to improve the effective SNR by enabling the receiver to reconstruct the original signal even when corrupted by noise-induced errors. The timing and structure of these codes are meticulously designed to optimize performance under noisy conditions.
Therefore, while the specific vocabulary may differ from one field to another, the underlying challenge of maximizing the desired signal relative to unwanted noise by carefully controlling the timing and repetition of measurements or excitations is a unifying principle. The insights gained from understanding the intricate relationship between TR and SNR in MRI can often provide valuable perspectives on optimizing signal detection and processing in a wide array of applications, underscoring the broad applicability of these fundamental concepts.
The Verdict: A Complex Interplay, Not a Simple Formula
Summarizing the Nuances of the Relationship
So, to circle back to our initial question: does increasing TR lead to an increase in SNR? The answer, as is often the case when dealing with intricate systems, is "it's not that simple." While a longer TR can indeed allow for a greater recovery of longitudinal magnetization in MRI, potentially resulting in a stronger signal and thus a higher SNR, this benefit is not guaranteed and comes with its own set of trade-offs. Longer TRs inherently mean longer scan times, which can increase the likelihood of motion artifacts and may not always be feasible in a busy clinical environment. Furthermore, the optimal TR is strongly influenced by the T1 relaxation times of the tissues being imaged; simply increasing TR beyond a certain point might not yield significant improvements in SNR and will only prolong the examination duration.
Conversely, shorter TRs enable faster image acquisition but often at the cost of reduced signal strength and a potentially lower SNR. The selection of an appropriate TR is a critical decision in the design of any MRI protocol, requiring a careful balancing act between achieving a sufficient signal-to-noise ratio, obtaining the desired image contrast, minimizing scan time, and meeting the specific objectives of the clinical examination. It's a multi-faceted optimization problem where numerous parameters interact in complex and sometimes counterintuitive ways. Therefore, a simple, direct statement about the relationship between increasing TR and increasing SNR would be an oversimplification of the underlying physical principles and practical considerations.
Instead of a direct, linear relationship, the influence of TR on SNR is indirect, mediated by its impact on the fundamental strength of the signal and, in certain scenarios, on the characteristics of the noise itself. Optimizing SNR requires a comprehensive understanding of the MRI system's capabilities, the inherent magnetic properties of the tissues being imaged, and the various sources of noise that can degrade image quality. It involves carefully selecting the TR in conjunction with other crucial imaging parameters, such as the flip angle of the RF pulse, the echo time, and the receiver bandwidth, to achieve the best possible image quality for the specific diagnostic task at hand. It's a testament to the sophisticated engineering and physics that underpin modern medical imaging technology.
In conclusion, while TR is undoubtedly a vital parameter that influences signal strength in MRI and consequently has an impact on SNR, simply increasing it does not automatically guarantee an improvement in the signal-to-noise ratio. The optimal TR is highly context-dependent, necessitating a nuanced understanding of the underlying principles and a careful consideration of the various trade-offs involved. It serves as a reminder that in the world of science and engineering, straightforward answers are often elusive, and true understanding lies in appreciating the intricate complexities and interdependencies of the systems we seek to analyze and optimize.
Frequently Asked Questions (FAQ)
Your Curiosities Addressed (Hopefully with a Touch of Clarity!)
Alright, let's tackle some of those lingering questions that might be swirling around in your mind like stray magnetic fields!
Q: So, if just turning up the TR isn't the magic fix for SNR, what actually works?
That's a sharp question! Think of it like trying to improve the sound quality of your favorite music; you can't just crank up the volume and expect it to sound better if the recording itself is poor. Optimizing SNR in MRI (and many other signal-sensitive areas) is a multi-pronged approach. It involves things like using stronger magnets (imagine a more organized and powerful chorus of protons!), employing optimized RF coils (better antennas to capture those faint signals more efficiently!), utilizing sophisticated pulse sequences (like a well-composed symphony to maximize signal return!), and even averaging multiple scans together (like taking several photos of the same scene and combining them to reduce random speckling!). TR is just one instrument in this complex but ultimately rewarding (high SNR!) performance.
Q: You mentioned T1 relaxation time. What is it exactly, and why should it matter to me?
Think of T1 relaxation time as the 'recovery' speed of our tiny proton magnets after they've been disturbed by the RF pulse. Different types of tissue have different recovery rates — some are quick to realign, while others take their sweet time. Knowing these T1 times is crucial because it guides our choice of TR. If our TR is too short for a particular tissue's T1, we're essentially trying to take a snapshot before the protons have had a chance to fully 'recover' their magnetization, resulting in a weaker signal. Choosing a TR that's somewhat aligned with the T1 time of the tissue we're interested in helps us obtain the strongest possible signal from it. So, yes, it's definitely something to consider if you're aiming for those clear, detailed images!
Q: What happens if the SNR is really low? Is it just a slightly fuzzy picture?
It's more significant than just a blurry image! Low SNR can obscure vital details, making it challenging for medical professionals to make accurate diagnoses. Imagine trying to read a faint inscription on a weathered stone in dim light. The uneven surface and poor lighting (the noise) make it difficult to discern the subtle markings (the signal). In MRI, low SNR can lead to missed findings or the necessity for repeat scans, which isn't ideal for anyone involved. That's why achieving an adequate SNR is so critical — it's about ensuring we can clearly see the information needed to make informed clinical decisions.